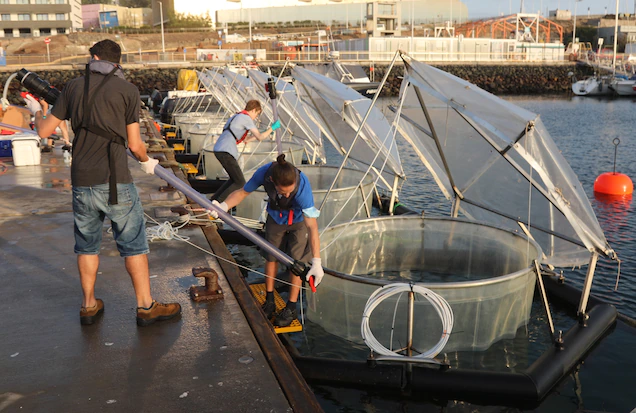
Before sunrise in a quiet village in Gran Canaria, an island off the coast of northwest Africa, a team of scientists shuffles along the port’s wooden boardwalk toward a row of nine containers floating in the ocean.
“Hurry up, it’s going to get light soon,” says one bleary-eyed researcher to another, as they submerge a hefty, cube-shaped device for measuring the activity of bioluminescent organisms into one container. “That will affect our readings.” The thermoplastic polyurethane “mesocosms,” filled with 8,000 liters of Canarian seawater mixed with varying amounts of limestone—a greyish, carbonate rock with high levels of alkaline—were part of the world’s first scientific field experiment with ocean alkalinity enhancement; research was completed in October. Many scientists hope that this little-examined process has the potential to turn the tide against climate change.
The goal of ocean alkalinity enhancement is to accelerate the carbon-absorbing weathering of rock, which naturally occurs as rainfall washes over land into waterways and eventually the ocean. Similar action happens through the gradual erosion of coastlines through wave action. “It’s continuously happening,” says Ulf Riebesell, a marine biologist at GEOMAR Helmholtz Center for Ocean Research in Kiel, Germany, who is leading the EU-funded team of 35 researchers. “The rock reacts with water, and during that reaction takes up CO2 from the atmosphere. The question is, can we significantly speed up that natural process? That’s what we’re simulating.”
“It’s a voyage into the unknown,” says Riebesell. “There’s so much we don’t yet know. But what’s certain is that alkalinity enhancement has enormous potential. And we need to test it now, because we’re running out of time to save the planet.”
Eye-opening potential
In theory, the natural process could be accelerated by depositing large amounts of pulverized silicate or carbonate rocks into the sea. Riebesell estimates that, while natural weathering sequesters one gigaton of CO2 per year, if enhanced weathering were scaled up massively, something in the ballpark of 100 gigatons of CO2 could be sequestered every year. Given that manmade CO2 emissions are 36 gigatons per year, the potential is eye opening. By stabilizing alkalinity levels, the process could at the same time help protect coral reefs against acidification. However, there are multiple causes for caution and concern.
While on paper the initial chemical process is straightforward, almost every other factor is unknown. How will biodiversity be impacted? Where should these minerals be deployed? Could there be unintended consequences? How much will it cost? Who decides if it should go ahead? And crucially, as Riebesell and his colleagues are testing, will it even work?
During the 33-day experiment, researchers studied samples from the mesocosms, which contained seawater that ranged from naturally occurring levels of alkalinity up to double that amount. About 45 parameters—from pH levels to plankton health—were analyzed at multiple laboratories at the Oceanic Platform of the Canary Islands and the Technology Park of the University of Las Palmas. A key objective of the ongoing research is to see whether adding alkaline minerals to seawater in this quantity will produce calcium carbonate, which would in turn release some CO2 and lessen the gains.
“Calcification might increase in response to alkalinity enhancement, which would lower the amount of CO2 being sequestered,” says Riebesell, whose team is studying the data in Germany. “But if the calcium stays in the water, and the alkalinity doesn’t lower back down, the CO2 stays in the ocean for good. That’s our hope.”
Rosalind Rickaby, a professor of biogeochemistry at Oxford University’s Department of Earth Sciences has for the past two years conducted lab research into whether calcification occurs, when alkalinity is increased, in cultures of single-celled organisms called coccolithophores and foraminifera. She’s seen positive signs.
“It’s small scale, so it’s difficult to come to a conclusion,” she says. “But the cells are being detrimentally affected by the alkalinity, which is a good thing. It’s evidence that by adding alkaline, you reduce CO2 levels, which the cells need for photosynthesis.”
Next year, in search of further clues, Riebesell’s team plans to carry out a follow-up study in the temperate and highly-productive waters of Norway, which stand in contrast to the waters of the Canary islands, which have little aquatic plant growth. The Norway test in May will involve much larger—50,000-liter—mesocosms, and so will provide insight into how more complex organisms such as fish are affected.
A herculean task
Even if the process does work, implementing ocean alkalinity enhancement to all of the oceans could prove a herculean task. Mining, milling, and transporting minerals would require an industry equivalent to coal mining, since sequestering one ton of CO2 would require between one and five tons of mineral, according to Riebsell. Then there’s the question of distribution: minerals could be deposited by ships, mixed with coastal sand, or even sprinkled on agricultural land. Each method would have various challenges, costs, and timescales.
“It’s feasible because there are enough minerals,” he says. “But it would be a huge undertaking. And should we even continue mining like this?”
The Gran Canaria test used limestone, which, although plentiful, doesn’t easily dissolve in water and must be mixed with concentrated CO2 solution, adding another level of logistics. Quicklime, a byproduct of the cement industry, dissolves easily and is abundant but requires burning of limestone to be produced, making it less effective at cutting emissions. The most promising option is olivine, a greenish silicate-based mineral that, pound for pound, sequesters twice as much CO2 as quicklime and four times as limestone. Olivine will be used in the Norway research.
A separate research program undertaken by California-based company Project Vesta also plans to deploy olivine in four field trials across the coastal waters of North Carolina, New York, India, and the northern Caribbean in the next few years. “It’s found all over the world, even on Hawaiian beaches,” says CEO Tom Green. “Its mining doesn’t require any chemicals, you just have to extract it, and you could use the world’s coal mining infrastructure.”
According to Project Vesta, just three tons of CO2 would be emitted in the process of ocean alkalinity enhancement using olivine for every 100 tons removed. Green says that makes it much more viable on a large scale than direct air capture—using machines to suck CO2 out of the atmosphere—since the latter requires a lot of energy. “We want to harness the power of oceans,” he says.
Are oceans a solution?
Oceans, which already soak up 90 percent of the planet’s excess heat and a quarter of CO2 emissions, are increasingly seen as the frontier of climate solutions, according to Jean-Pierre Gattuso, a professor at the Villefranche Oceanography Laboratory in Nice, France. In contrast, land-based efforts such as reforestation only remove a relatively small amount of CO2 from the atmosphere, can only replace what’s already been emitted, and are unlikely to produce permanent gains, since forests can be logged or burned.
“Our emissions reductions simply aren’t fast enough,” says Gattuso, who in January published a policy briefing in Frontiers in Climate on ocean-based technologies such as alkalinity enhancement, iron fertilization (using iron to stimulate phytoplankton growth), and artificial upwelling (circulating nutrient-rich deep waters upward). “So what’s needed are technologies that remove CO2 from the air. In that regard, the ocean quite obviously has the most potential.”
Turning that potential into reality is another matter, and experts say there are tough criteria that must be met: verifiability, although impact can take years to prove; scalability, which would need to be enormous; economic feasibility, due to the required scale; permanence, which is almost impossible to guarantee; accountability, even though a governance structure doesn’t exist; and, of course, environmental soundness.
“Alkalinity enhancement has the greatest potential [of these ocean-based solutions],” says Riebsell. “But more research has to be performed. We need years to reach a point where we understand the risks to some extent. We will never fully understand those risks.”
Time is running out
Some critics fear large-scale manipulation of the Earth’s natural systems will lead to unpredictable, damaging outcomes, like the introduction of European rabbits to Australia in the 19th century, which devastated native species as the population exploded from just 13 to 200 million in about half a century. Others warn that if the idea of a quick fix—allowing the world to return to business as usual—is promoted, it will discourage climate action and regalvanize the fossil fuel industry.
For Riebesell, who has studied the impacts of climate change on oceans—from rapid warming to acidification and de-oxygenation—for over a decade, those concerns are valid, but as time runs out for humanity to avert an irreversible climate disaster, he sees little choice.
“Ten years down the line we will have to make a decision about what we employ to reach our climate targets,” he says. “So we need to know which of these options are really scalable, the risks, the costs, and the impacts. Avoiding this research will not make the problem go away. We must find a solution.”